Abstract
Background: The relationship between assisted reproductive technology (ART) and childhood cancer risk has been widely debated. Previous meta-analyses did not adequately account for the impact of infertility, and this study addresses this gap.
Objective: Our primary objective was to assess the relative risk (RR) of childhood cancer in infertile populations using ART versus non-ART offspring, with a secondary focus on comparing frozen embryo transfer (FET) and fresh embryo transfer (fresh-ET).
Methods: A literature review was conducted through PubMed, Embase, Cochrane, and Web of Science, with a cutoff date of July 10, 2024. The study was registered with the International Platform of Registered Systematic Review and Meta-Analysis Protocols (INPLASY 202470119). Inclusion criteria were based on the PICOS (Population, Intervention, Comparison, Outcomes, and Study Design) framework: infertile or subfertile couples (population), ART interventions (in vitro fertilization [IVF], intracytoplasmic sperm injection [ICSI], FET, and fresh-ET), non-ART comparison, and childhood cancer risk outcomes. Data abstraction focused on the primary exposures (ART vs non-ART and FET vs fresh-ET) and outcomes (childhood cancer risk). The risk of bias was assessed using the Newcastle-Ottawa Quality Assessment Scale, and the evidence quality was evaluated with the Grading of Recommendations Assessment, Development, and Evaluation (GRADE). Pooled estimates and 95% CIs were calculated using random effects models.
Results: A total of 18 studies were included, published between 2000 and 2024, consisting of 14 (78%) cohort studies and 4 (22%) case-control studies, all of which were of moderate to high quality. The cohort studies had follow-up periods ranging from 3 to 18 years. Compared with non-ART conception, ART conception was not significantly associated with an increased risk of childhood overall cancer (RR 0.95, 95% CI 0.71‐1.27; GRADE quality: low to moderate). Subgroup analyses of IVF (RR 0.86, 95% CI 0.59‐1.25), ICSI (RR 0.76, 95% CI 0.26‐2.2), FET (RR 0.98, 95% CI 0.54‐1.76), and fresh-ET (RR 0.75, 95% CI 0.49‐1.15) showed similar findings. No significant differences were found for specific childhood cancers, including leukemia (RR 0.99, 95% CI 0.79‐1.24), lymphoma (RR 1.22, 95% CI 0.64‐2.34), brain cancer (RR 1.22, 95% CI 0.73‐2.05), embryonal tumors (RR 1, 95% CI 0.63‐1.58), retinoblastoma (RR 1.3, 95% CI 0.73‐2.31), and neuroblastoma (RR 1.02, 95% CI 0.48‐2.16). Additionally, no significant difference was observed in a head-to-head comparison of FET versus fresh-ET (RR 0.99, 95% CI 0.86‐1.14; GRADE quality: moderate).
Conclusions: In conclusion, this study found no significant difference in the risk of childhood cancer between offspring conceived through ART and those conceived through non-ART treatments (such as fertility drugs or intrauterine insemination) in infertile populations. While infertility treatments may elevate baseline risks, our findings suggest that whether individuals with infertility conceive using ART or non-ART methods, their offspring do not face a significantly higher risk of childhood cancer. Further research, especially comparing infertile populations who conceive naturally, is needed to better understand potential long-term health outcomes.
Trial Registration: INPLASY 202470119; https://inplasy.com/?s=202470119
doi:10.2196/65820
Keywords
Introduction
Over the last century, global fertility rates have significantly declined, and it is projected that by 2060, fertility will fall below replacement levels [
, ]. This trend is closely linked to an increase in infertility, which can be caused by factors such as ovulation disorders, tubal abnormalities, uterine issues, and sperm abnormalities [ ]. Assisted reproductive technology (ART) has helped many infertile couples achieve parenthood. Since ART’s introduction in 1978, over 10 million children have been born using this technology [ ], with approximately 1 million children conceived via ART each year. As ART usage increases, monitoring the long-term health risks associated with it, particularly childhood cancer, becomes crucial [ ].The relationship between ART and childhood cancer has been widely studied, but the results remain controversial due to inconsistent findings [
, ]. One of the key reasons for this inconsistency is the use of different reference groups. Few studies distinguish between children born to parents with infertility and those born to parents who conceived naturally [ , ]. It is essential to differentiate the effects of parental infertility from those of ART treatment, particularly given the challenge of small sample sizes in many studies. Furthermore, most studies are conducted within a single health care system or region, which limits their ability to fully assess cancer risk in offspring conceived through ART.Previous reviews and meta-analyses have not adequately addressed infertility as a factor, possibly due to the limited availability of relevant studies [
- ]. However, recent large national cohort studies have compared offspring of parents with infertility with controls, and follow-up periods have extended beyond 10 years [ - ]. Given these advances, we conducted a systematic review and meta-analysis to assess the relative risk (RR) of childhood cancer in ART versus non-ART offspring in infertile populations and to compare frozen embryo transfer (FET) with fresh embryo transfer (fresh-ET). This study provides new insights into the relationship between ART modalities and pediatric cancer risk, which could help guide clinical ART fertility treatments.Methods
Overview
This study was retrospectively registered with the International Platform of Registered Systematic Review and Meta-Analysis Protocols (INPLASY 202470119). The systematic review followed the PRISMA (Preferred Reporting Items for Systematic Reviews and Meta-Analyses) guidelines and included all published articles on ART exposure and childhood cancer risk in the offspring of parents with infertility [
].Search Strategy and Inclusion Criteria
We conducted a systematic literature search with a deadline of July 10, 2024, using PubMed, Embase, Cochrane, and Web of Science. The electronic search strategy was initially developed by the author (GS) and subsequently reviewed by the author with extensive search experience (MQC). We first tested the search by adapting it for each database and validating it against previously published meta-analyses on relevant topics to ensure the comprehensiveness of our approach. The validated search strategy was implemented simultaneously across each database on July 10, 2024, using the search terms “ART,” “children,” “cancer,” and “risk.” The detailed Boolean expressions of the search strategy are presented in
.The inclusion criteria were constructed using the PICOS (Population, Intervention, Comparison, Outcomes, and Study Design) framework:
- Population: infertile or subfertile couples.
- Intervention: ART, including in vitro fertilization (IVF), intracytoplasmic sperm injection (ICSI), FET, and fresh-ET.
- Comparison: non-ART, defined as infertile or subfertile couples who did not conceive through ART but may have conceived naturally or with induced ovulation induction (OI) or intrauterine insemination (IUI).
- Outcomes: risk of childhood cancer, including overall childhood cancers and specific types such as leukemia, lymphomas, brain cancer, embryonal tumors, retinoblastoma, and neuroblastoma.
- Study design: randomized controlled trials and observational studies (eg, cohort or case-control studies).
Studies lacking sufficient data to calculate RR estimates and their 95% CIs were excluded. Additionally, conference abstracts, reviews, non-English articles, duplicate data, and non–peer-reviewed publications were excluded.
Study Selection
On July 10, 2024, 2 researchers (CQZ and RL) conducted literature searches, reviewed the results, and imported them into Endnote X8 (Clarivate Analytics). CQZ was responsible for deduplication and the initial screening of studies, while RL reviewed CQZ’s selections. Both researchers then independently performed further screening based on the predefined inclusion criteria. Any disagreements were resolved through discussion between CQZ and RL. If a consensus could not be reached, a third researcher, GS, was consulted.
Data Extraction
Data extraction was carried out by CQZ using a prespecified and tested form in Microsoft Excel. RL then reviewed the extracted data for accuracy. The information extracted included the first author, year of publication, age at follow-up, study design, study timeframe, country, data source, duration of follow-up, type of cancer reported, ART type (IVF, ICSI, FET, or fresh-ET), and case-control or exposure-nonexposure data. If any data were missing, the authors were contacted to obtain the necessary information.
Quality Assessment and Risk of Bias
The Newcastle-Ottawa Quality Assessment Scale (NOS) was used for the quality assessment of the included studies [
]. Two authors (CQZ and RL) independently conducted the NOS evaluation, and any disagreements were resolved through discussions with the corresponding author or GS. Studies were categorized into low (total score ≥7), moderate (total score 5‐6), and high (total score ≤4) risk of bias.Publication bias for the primary outcomes, such as ART versus non-ART and FET versus fresh-ET, was assessed using funnel plots and the Egger test. If the points on the funnel plot were symmetrically distributed, it indicated no or low bias; asymmetry suggested the presence of publication bias. The Egger test was performed to quantitatively assess publication bias, with a P<.05 indicating significant bias. Sensitivity analyses were conducted for the primary outcomes.
Data Analysis and Synthesis
The RR and 95% CIs were chosen to assess the association between ART and childhood cancer in infertile offspring. Outcomes were combined using the DerSimonian and Laird random effects model [
]. All analyses were visualized using Stata 17 statistical software, and in meta-analyses, P<.05. Heterogeneity was analyzed using the I2 statistic. A high degree of heterogeneity was indicated if the I2 value was greater than 50%. Subgroup analyses were conducted based on the following four criteria: (1) continents, (2) duration of follow-up, (3) reported cancer type, and (4) operational versus nonoperational. Subgroup differences were assessed using the Q test, and statistical significance was defined as a P<.05. Regardless of the level of heterogeneity, a random-effects model was consistently applied to ensure the robustness of the analysis across different study designs and populations. Sensitivity analyses were performed by excluding each study individually.Quality of Evidence
GRADE (Grading of Recommendations Assessment, Development, and Evaluation) is a systematic approach for evaluating the quality of evidence by assessing 5 domains: methodological limitations (eg, risk of bias), heterogeneity of results (eg, inconsistency), generalizability of findings (eg, indirectness), precision of estimates, and risk of publication bias [
]. The overall certainty of the evidence is categorized into 4 levels, ranging from high to very low.Results
Search Results and Study Characteristics
A total of 2505 articles were obtained from the systematic search, of which 302 (12.06%) were duplicates. We screened the titles and abstracts to exclude 2167 (86.51%) articles that did not meet the eligibility criteria and subsequently removed them. The full manuscripts of the 36 articles were screened to exclude 18 (50%) articles that did not meet the eligibility criteria. These included different papers by the same authors with duplicate data. Data that did not involve subfertile offspring were excluded. A total of 18 studies [
- , - ] were thus included in this review. The NOS quality of the included studies was either moderate or high (Table S1 in ) [ - , - ]. The PRISMA flowchart depicts the article screening process ( ).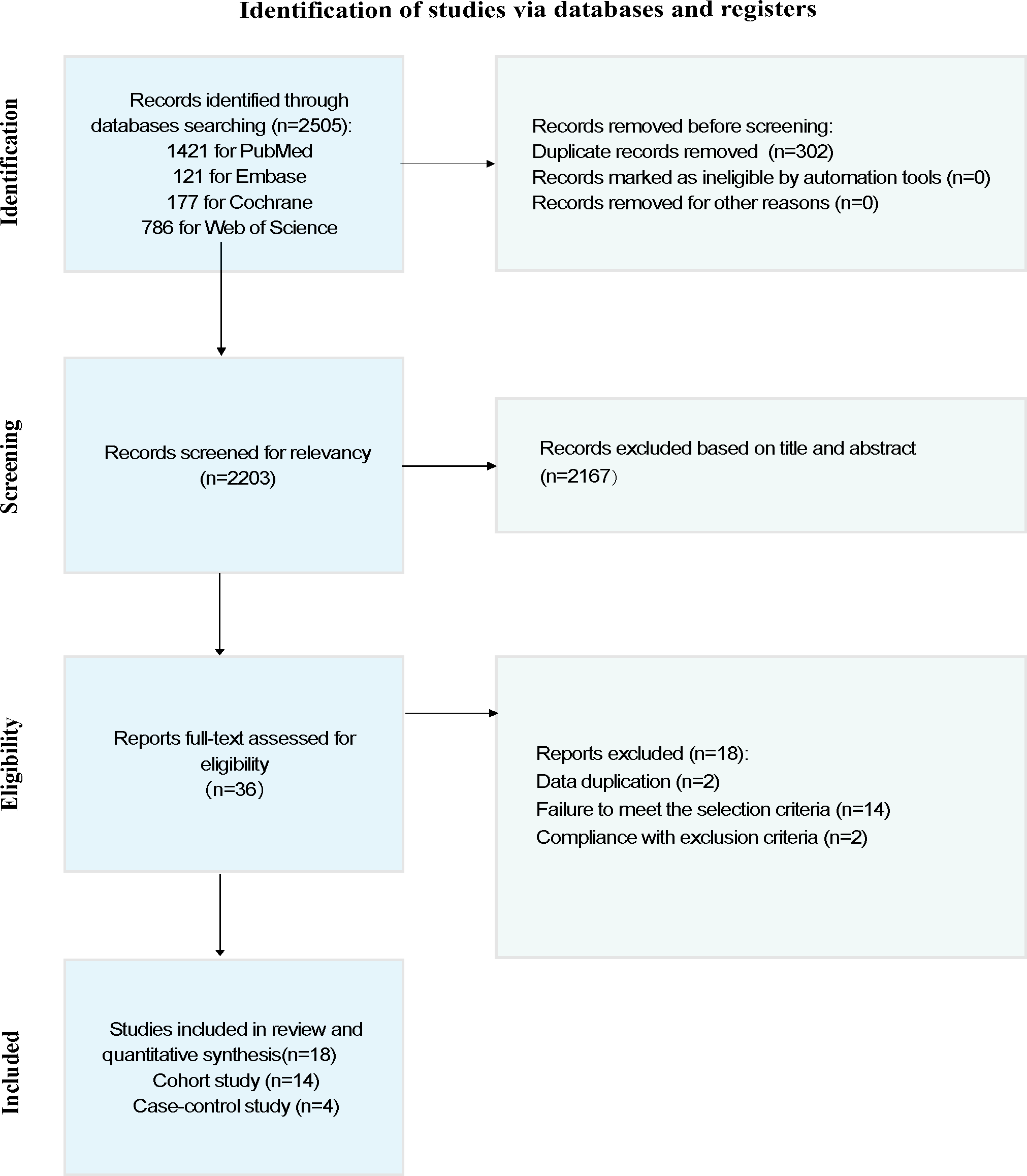
Of the 18 included studies, 14 (77%) were cohort studies [
- , - ] and 4 (22%) were case-control studies [ - ]. All cohort studies reported overall cancer occurrence risk, while the 4 case-control studies focused only on specific types of cancer, including retinoblastoma, leukemia, and neuroblastoma. All studies were published in English and covered multiple countries and regions, including Australia, Israel, Denmark, the United States, France, Finland, Sweden, the Netherlands, Taiwan, the United Kingdom, and Norway. The studies were published across nearly 2 decades, with the earliest study published in 2000 [ ] and the most recent study published in 2024 [ ]. Most cohort studies had follow-up durations ranging from 3 to 18 years, with the shortest follow-up period being 3 years [ ] and the longest extending to 18 years [ ].Of the 18 studies, 10 (56%) [
- , - ] compared ART with non-ART and 11 (61%) [ , , - , - ] compared FET with fresh-ET. Of the 10 studies comparing ART with non-ART, 6 (60%) were cohort studies [ - ] involving 480,852 ART patients and 716,144 non-ART patients. Four (4/10, 40%) [ - ] were case-control studies involving 563 ART patients and 1521 non-ART patients. Of the 18 studies, 11 (61%) comparative studies of FET versus fresh-ET were cohort studies involving 176,800 FET patients and 723,327 fresh-ET patients.Comparison of Childhood Overall Cancer Risk by ART Conception and Non-ART Conception
Of the 18 studies, 6 (33%) studies have compared the risk of childhood overall cancer in offspring of ART versus non-ART conceptions (Table S2 in
) [ - ]. The results showed that there was no significant increase in the risk of childhood overall cancer in ART-conceived offspring compared with non-ART conception (RR 0.95, 95% CI 0.71‐1.27; I2=82%) ( ). A high degree of heterogeneity was observed. We performed subgroup analyses based on continent, follow-up duration, reported cancer types, and whether artificial insemination procedures were involved. No significant differences were observed within the subgroups ( ). However, when the non-ART control group was defined as nonoperational (ie, using only OI or fertility drugs), the RR for childhood overall cancer in the ART group was 1.23 (95% CI 0.98‐1.54). Based on the GRADE evidence quality assessment, the quality of the comparison between ART and non-ART was rated as “low to moderate” due to serious risk of bias and inconsistency (Table S3 in ). The Egger test did not detect significant publication bias (P=.66), and the adjusted RR was 0.812 (95% CI 0.549‐1.074), indicating robust results (Figure S1 in ).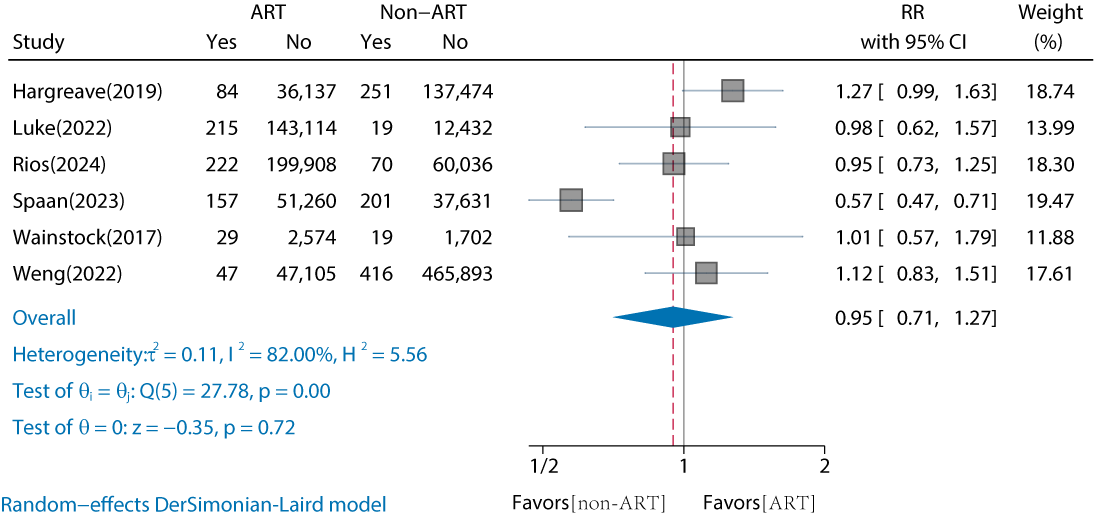
Studies, n | ART, n | Non-ART, n | RR | (95% CI)I2 (%) | PHeterogeneity | PBetween groups | ||
Overall | 6 | 480,852 | 716,144 | 0.95 (0.71‐1.27) | 82 | <.001 | — | |
Continents | .73 | |||||||
Asian | 2 | 49,755 | 468,030 | 1.09 (0.84‐1.43) | 0 | .76 | — | |
Europe | 3 | 287,768 | 235,663 | 0.88 (0.54‐1.43) | 91.83 | <.001 | — | |
North America | 1 | 143,114 | 12,451 | 0.98 (0.62‐1.57) | — | — | — | |
Duration of follow-up (years) | .69 | |||||||
≤10 | 3 | 390,611 | 538,866 | 1.02 (0.84‐1.22) | 0 | .73 | — | |
>10 | 3 | 90,241 | 177,278 | 0.89 (0.49‐1.62) | 91.61 | <.001 | — | |
Reported cancers type | .84 | |||||||
Neoplasm | 1 | 2603 | 1721 | 1.01 (0.57‐1.79) | — | — | — | |
Overall cancer | 5 | 478,249 | 714,423 | 0.94 (0.68‐1.31) | 85.5 | <.001 | — | |
Operational versus nonoperational | .10 | |||||||
Non-ART (nonoperational) | 2 | 38,711 | 139,176 | 1.23 (0.98‐1.54) | 0 | .47 | — | |
Non-ART (operational) | 4 | 441,387 | 575,992 | 0.87 (0.61‐1.22) | 82.08 | <.001 | — |
aART: assisted reproductive technology.
bRR: relative risk.
cNot applicable.
dWe set non-ARTs that only use fertility drugs or ovulation induction as a nonoperational factor, and those that involve artificial insemination or intrauterine insemination operations as an operational factor.
In addition, we compared IVF, ICSI, FET, and fresh-ET conceptions with non-ART conceptions separately. The results showed no significant differences between either (P≥.05). The corresponding RRs were for IVF (RR 0.86, 95% CI 0.59‐1.25; I2=70.18%), ICSI (RR 0.76, 95% CI 0.26‐2.2; I2=94.61%), FET (RR 0.98, 95% CI 0.54‐1.76; I2=83.18%), and fresh-ET (RR 0.75, 95% CI 0.49‐1.15; I2=81.85%) (Figures S1-S4 in
) [ - , ].Comparison of Childhood Overall Cancer Risk by FET Conception and Fresh-ET Conception
Of the 18 studies, 11 (61%) cohort studies compared the risk of childhood overall cancer in FET versus fresh-ET conceived offspring (Table S4 in
) [ , , - , - ]. The results showed no significant increase in the risk of childhood overall cancer for FET-conceived offspring compared to fresh-ET (RR 0.99, 95% CI 0.86‐1.14; ). The interstudy heterogeneity was low (I2=24.45%). Subgroup analyses by continent, follow-up duration, and cancer type revealed no significant differences ( ). Funnel plots and the Egger test indicated no publication bias (t=0.53, P=.61; adjusted RR 0.98, 95% CI 0.856‐1.125; Figure S2 in ). Based on the GRADE assessment, the quality of the comparison between FET and fresh-ET was rated as “moderate” due to a serious risk of bias (Table S3 in ).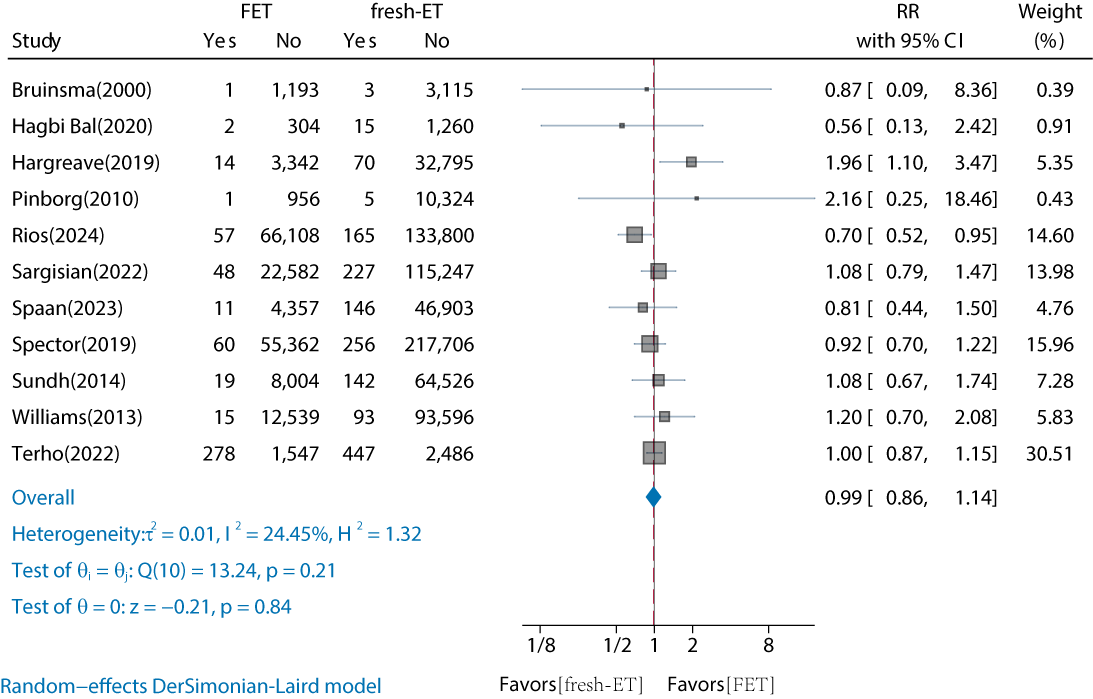
Studies, n | RR | (95% CI)I2 (%) | PHeterogeneity | PBetween groups | ||
Overall | 11 | 0.99 (0.86‐1.14) | 24.45 | .21 | — | |
Continents | .21 | |||||
Asian | 1 | 0.56 (0.13‐2.42) | — | — | — | |
Europe | 8 | 1.02 (0.84‐1.23) | 43.58 | .09 | — | |
North America | 1 | 0.92 (0.7‐1.22) | — | — | — | |
Oceania | 1 | 0.87 (0.09‐8.36) | — | — | — | |
Duration of follow-up (years) | .37 | |||||
≤10 | 8 | 0.92 (0.79‐1.08) | 0 | .47 | — | |
>10 | 3 | 1.13 (0.75‐1.72) | 64.27 | .06 | — | |
Reported cancers type | .94 | |||||
Neoplasm | 2 | 0.99 (0.87‐1.14) | 0 | .44 | — | |
Overall cancer | 9 | 1 (0.82‐1.23) | 36.27 | .13 | — |
aFET: frozen embryo transfer.
bfresh-ET: fresh embryo transfer.
cRR: relative risk.
dNot applicable.
Comparison of Childhood-Specific Cancer Risk by ART Conception and Non-ART Conception
In total, 10 studies compared the risk of childhood-specific cancer in the offspring of ART versus non-ART conceptions (Tables S5 and S6 in
) [ - , - ]. The main studies included 6 cohort studies and 4 case-control studies. The results showed that none of the ART-conceived offspring had a significantly increased risk of childhood-specific cancer compared to non-ART conception (P≥.05). The main ones included leukemia (RR 0.99, 95% CI 0.79‐1.24; I2=12.79%), lymphoma (RR 1.22, 95% CI 0.64‐2.34; I2=54.76%), brain cancer (RR 1.22, 95% CI 0.73‐2.05; I2=45.79%), embryonal tumors (RR 1, 95% CI 0.63‐1.58; I2=0%), retinoblastoma (RR 1.3, 95% CI 0.73‐2.31; I2=0%), and neuroblastoma (RR 1.02, 95% CI 0.48‐2.16; I2=0%) ( ).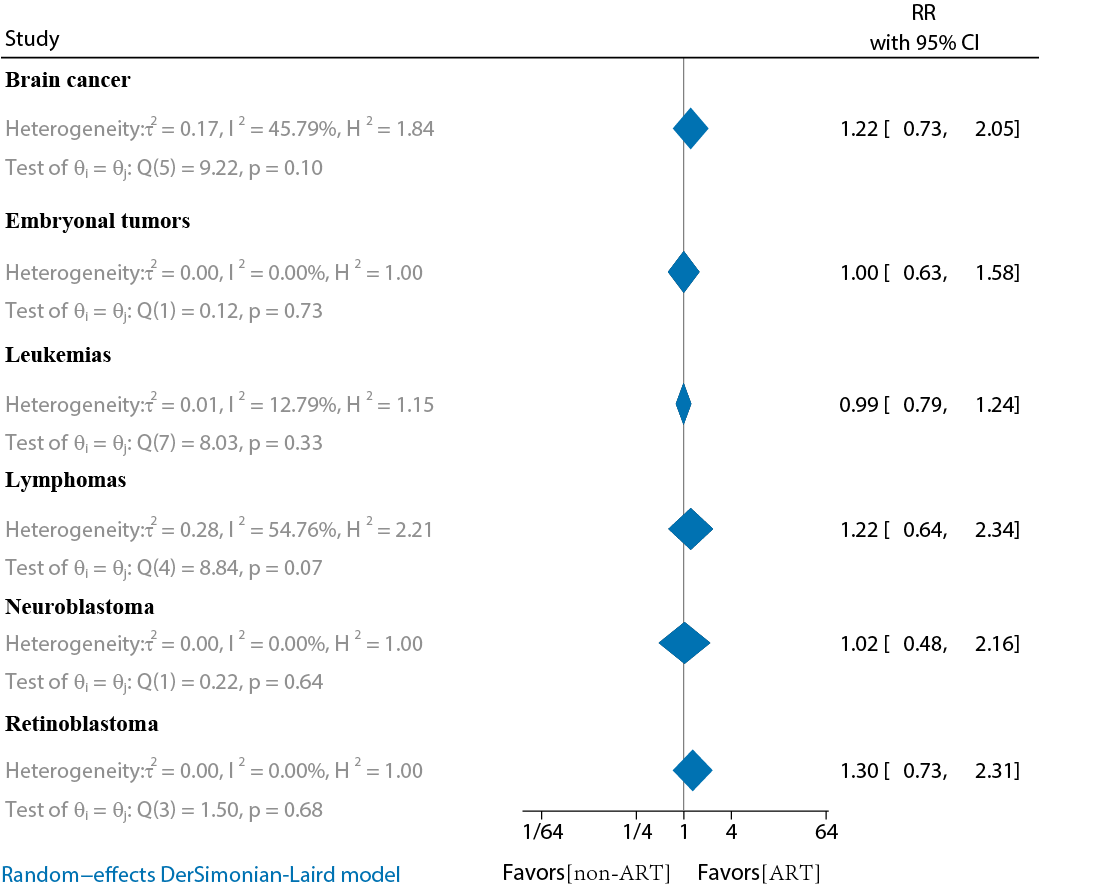
Sensitivity Analysis
To explore the stability of the meta-analysis results, sensitivity analyses were performed by excluding each study individually. The results demonstrated the robustness of findings for both ART versus non-ART conception and FET versus fresh-ET conception regarding childhood cancer risk. The results remained consistent even after excluding the study by Spaan et al [
] (RR 1.1, 95% CI 0.95‐1.26; Figure S3 in ) [ - , - ].Discussion
Principal Findings
This study, to our knowledge, is the first systematic review and meta-analysis to use infertile or subfertile populations as the reference group. We found no significant increase in childhood overall cancer risk in ART-conceived offspring compared to non-ART. This result was consistent across different ART methods, including IVF, ICSI, FET, and fresh-ET. Furthermore, no significant differences were observed between FET and fresh-ET in terms of childhood cancer risk. Despite the heterogeneity of the studies included, the results were robust across sensitivity analyses, supporting the stability and reliability of our findings.
Comparison to Prior Work
To date, 6 meta-analyses have examined the association between ART fertility treatments and childhood cancer risk. The meta-analyses by Wang et al [
], Chiavarini et al [ ], and Hargreave et al [ ] found a significant correlation between ART and childhood cancer risk, while those by Raimondi et al [ ], Gilboa et al [ ], and Zhang et al [ ] did not support such an association. Recent large-scale cohort studies have yet to reach a consensus on this issue. Some studies report a significant association between ART conception and increased childhood cancer risk [ , , , , ]. For instance, a large Nordic study by Sargisian et al [ ], which included data from Denmark, Norway, Sweden, and Finland, found a significantly increased risk of childhood cancer in ART-conceived offspring compared to naturally conceived offspring (RR 1.13, 95% CI 1.01‐1.26). However, other studies have not observed this association [ , , ]. The inconsistencies in these findings may be due to differences in control group selection, sample size, and follow-up duration [ , , , ].Recent evidence suggests that epigenetic changes may play a key role in causing infertility, rather than being simply a result of fertility treatments [
, ]. Couples experiencing infertility may already have a higher risk of epigenetic defects in their gametes, which fertility treatments have only helped to reveal [ ]. The present meta-analysis provided new insights, and we selected an appropriate control group to eliminate the effects of infertile or subfertile. We included 6 large cohort studies involving 480,852 ART conceptions and 716,144 non-ART conceptions. In our analysis of the non-ART control group, we performed subgroup analysis by categorizing it into operational (IUI or artificial insemination) and nonoperational (OI or fertility drugs) groups. When the non-ART control group was defined as nonoperational, the RR for childhood overall cancer in the ART group increased to 1.23 (95% CI 0.98‐1.54), approaching the statistical significance threshold. The review by Berntsen et al [ ] suggested that a scientific control group should consist of children of low-fertility parents who conceived naturally. However, obtaining such controls is challenging because they are rarely included in registry data. Additionally, it was noted that children born through fertility measures, such as ovarian stimulation or IUI, could also serve as suitable controls. Due to limitations in current published studies, we focused on studies with the latter control group approach. Future research should aim to include offspring born to low-fertility parents who conceived naturally to better understand the long-term effects of both infertility and fertility treatments.ICSI has become increasingly common worldwide, with approximately one-third of fresh ART cycles using conventional IVF and two-thirds using ICSI [
, ]. Despite the invasive nature of ICSI and ongoing concerns about the health of children born through this method, our meta-analysis, which included 2 eligible studies, showed that the risk of childhood overall cancer in ICSI-conceived offspring was not significantly higher compared to non-ART offspring (RR 0.76, 95% CI 0.26‐2.2; I2=94.61%). However, there was considerable heterogeneity between the studies, and further research is needed to confirm the long-term safety of ICSI regarding childhood cancer risk.FET accounts for 32.6% of all ART treatment cycles in Europe, showing a clear increasing trend [
]. Large cohort studies and meta-analyses have provided short-term health data on FET, such as perinatal outcomes [ - ]. Compared to singletons born after fresh-ET, infants born after FET generally have higher birth weights and a higher risk of LGA (large for gestational age) in suprapregnant children but lower perinatal mortality. Singletons born after FET are at an increased risk of LGA and preterm labor compared to naturally conceived offspring [ ]. However, data on the long-term health of FET offspring are limited. Studies comparing FET with naturally conceived offspring suggest an increased cancer risk in FET-conceived children [ , ].In our meta-analysis, which included 4 large cohort studies with infertile populations as the comparison group, we found no significant increase in childhood overall cancer risk in FET-conceived offspring compared to non-ART offspring (RR 0.98, 95% CI 0.54‐1.76; I2=83.18%). Given the high heterogeneity, these results should be interpreted with caution. Additionally, to our knowledge, no meta-analyses have compared childhood cancer risk between FET and fresh-ET conceived offspring. Our analysis, which included 11 cohort studies with 176,800 FET-conceived and 723,327 fresh-ET-conceived individuals, found no significant difference in cancer risk between the 2 groups (RR 0.99, 95% CI 0.86‐1.14), with low heterogeneity (I2=24.45%). No significant bias was found, and sensitivity analyses confirmed the stability of the results.
Several studies have explored the association between ART conception and specific childhood cancers, including leukemia [
, ], lymphoma [ ], hepatoblastoma [ , ], retinoblastoma [ ], and central nervous system tumors [ ]. A 2013 meta-analysis by Hargreave et al [ ] reported an increased risk of cancers such as leukemia (RR 1.65), neuroblastoma (RR 4.04), and retinoblastoma (RR 1.62). A 2019 meta-analysis by Chiavarini et al [ ] found that ART significantly increased the risk of hematological neoplasms (odds ratio [OR] 1.3, 95% CI 1.08‐1.58) and neurological cancers (OR 1.21, 95% CI 1.01‐1.46). Furthermore, a 2020 study by Zhang et al [ ] showed a significantly increased risk of hematologic cancers (RR 1.39), other solid tumors (RR 1.57), and leukemia (RR 1.31). Leukemia is one of the most common childhood cancers and a leading cause of death in children, followed closely by lymphoma and central nervous system tumors [ ]. Although several studies have suggested that ART is associated with an increased risk of childhood leukemia, most compared ART offspring with those conceived naturally [ , , , , ]. In contrast, our analysis included 8 studies on leukemia, 3 of which had follow-up durations of more than 10 years, and 2 were case-control studies. The results showed no significant increase in leukemia risk in ART offspring compared to non-ART offspring (RR 0.99, 95% CI 0.79‐1.24; I2=12.79%). When cohort studies were analyzed separately, the results remained unchanged (RR 1.1, 95% CI 0.87‐1.4; I2=4.05%). Additionally, no significant differences were found in further analyses of other specific childhood cancers, including lymphoma (RR 1.22, 95% CI 0.64‐2.34), brain cancer (RR 1.22, 95% CI 0.73‐2.05), embryonal tumors (RR 1, 95% CI 0.63‐1.58), retinoblastoma (RR 1.3, 95% CI 0.73‐2.31), and neuroblastoma (RR 1.02, 95% CI 0.48‐2.16).Strengths and Limitations
Strengths
One strength of this study is the use of a more appropriate control group, that is, infertile or subfertile populations, which enhances the reliability of the comparisons and helps address the risk of epigenetic defects associated with infertility. Additionally, our estimates were not significantly affected by recall bias, which is common in case-control studies [
]. Parents of children with cancer may be more likely to recall past events, potentially overestimating cancer risk. We combined 4 eligible case-control studies, mainly focusing on specific cancer types, and performed subgroup analyses, showing no significant differences across subgroups. Furthermore, large cohort studies with long-term and comprehensive data were recently included, reducing the risks of selection, attrition, and recall bias, while providing more opportunities to observe rare cancer exposures, thus enhancing the credibility of the findings.Limitations
First, the sample size of infertile or subfertile populations was small. Despite a comprehensive search, the limited number of studies, especially on ICSI and FET offspring, may reduce confidence in the findings. Larger sample sizes are needed in future research for greater statistical power. Second, this meta-analysis did not classify the non-ART control group further. The lack of distinction between naturally conceived offspring from low-fertility parents and those conceived through ovarian stimulation or IUI may introduce confounding, affecting the cancer risk baseline. Future studies should differentiate these groups to better assess ART’s impact on childhood cancer risk. Third, while some studies reported male infertility, gender-specific analyses were not performed, preventing separate calculations for male and female infertility. Future studies should address this to explore gender-specific effects on offspring health after ART. Fourth, the included studies used raw data without adjusting for factors like age, gender, birth order, socioeconomic status, and history of abortion. This lack of adjustment may affect result interpretation. Future studies should include adjusted data for more accurate conclusions.
Conclusions
This study found no significant difference in the risk of childhood cancer between offspring conceived through ART and those conceived through non-ART treatments (such as fertility drugs or OI/IUI) in infertile populations. While infertility treatments may elevate baseline risks, our findings suggest that whether individuals with infertility conceive using ART or non-ART methods, their offspring do not face a significantly higher risk of childhood cancer. Further research, especially comparing infertile populations who conceive naturally, is needed to better understand potential long-term health outcomes.
Acknowledgments
This study was funded by the Yunnan Province Science and Technology Department (no. 202201AY070001-294) and the Science and Technology Popularization Program (no. 202404AM350308).
Data Availability
All data generated or analyzed during this study are included in this published article and its supplementary files.
Authors' Contributions
Conceptualization: GS, MQC
Data curation: CQZ, RL, GS, ZPB
Formal analysis: CQZ, RL, GS
Writing – original draft: GS, MQC
Writing – review & editing: GS, MQC, CQZ, RL
Conflicts of Interest
None declared.
Literature search strategy.
DOC File, 54 KBSummary tables.
DOC File, 160 KBFunnel charts and sensitivity analyses.
DOC File, 138 KBForest plot of cancer risk in offspring of different types of ART (IVF, ICSI, FET, and fresh-ET). ART: assisted reproductive technology; FET: frozen embryo transfer; fresh-ET: fresh embryo transfer; ICSI: intracytoplasmic sperm injection; IVF: in vitro fertilization.
DOC File, 49 KBPRISMA (Preferred Reporting Items for Systematic Reviews and Meta-Analyses) checklist.
DOCX File, 25 KBReferences
- Ono M, Kuji N, Ueno K, Kojima J, Nishi H. The long-term outcome of children conceived through assisted reproductive technology. Reprod Sci. Mar 2024;31(3):583-590. [CrossRef]
- Ishihara O, Jwa SC, Kuwahara A, et al. Assisted reproductive technology in Japan: a summary report for 2018 by the Ethics Committee of the Japan Society of Obstetrics and Gynecology. Reprod Med Biol. Jan 2021;20(1):3-12. [CrossRef] [Medline]
- Carson SA, Kallen AN. Diagnosis and management of infertility: a review. JAMA. Jul 6, 2021;326(1):65-76. [CrossRef] [Medline]
- Pinborg A, Wennerholm UB, Bergh C. Long-term outcomes for children conceived by assisted reproductive technology. Fertil Steril. Sep 2023;120(3 Pt 1):449-456. [CrossRef] [Medline]
- Berntsen S, Söderström-Anttila V, Wennerholm UB, et al. The health of children conceived by ART: “the chicken or the egg?”. Hum Reprod Update. Mar 1, 2019;25(2):137-158. [CrossRef] [Medline]
- Graham ME, Jelin A, Hoon AH Jr, Wilms Floet AM, Levey E, Graham EM. Assisted reproductive technology: short‐ and long‐term outcomes. Develop Med Child Neuro. Jan 2023;65(1):38-49. [CrossRef]
- Stern JE, Farland LV, Hwang SS, et al. Assisted reproductive technology or infertility: what underlies adverse outcomes? Lessons from the Massachusetts Outcome Study of Assisted Reproductive Technology. F S Rev. Oct 2022;3(4):242-255. [CrossRef] [Medline]
- Zhang S, Luo Q, Meng R, Yan J, Wu Y, Huang H. Long-term health risk of offspring born from assisted reproductive technologies. J Assist Reprod Genet. Mar 2024;41(3):527-550. [CrossRef] [Medline]
- Raimondi S, Pedotti P, Taioli E. Meta-analysis of cancer incidence in children born after assisted reproductive technologies. Br J Cancer. Oct 2005;93(9):1053-1056. [CrossRef]
- Hargreave M, Jensen A, Toender A, Andersen KK, Kjaer SK. Fertility treatment and childhood cancer risk: a systematic meta-analysis. Fertil Steril. Jul 2013;100(1):150-161. [CrossRef] [Medline]
- Gilboa D, Koren G, Barer Y, et al. Assisted reproductive technology and the risk of pediatric cancer: a population based study and a systematic review and meta analysis. Cancer Epidemiol. Dec 2019;63:101613. [CrossRef] [Medline]
- Wang T, Chen L, Yang T, et al. Cancer risk among children conceived by fertility treatment. Int J Cancer. Jun 15, 2019;144(12):3001-3013. [CrossRef] [Medline]
- Zhang Y, Gao R, Chen H, et al. The association between fertility treatments and the incidence of paediatric cancer: a systematic review and meta-analysis. Eur J Cancer. Oct 2020;138:133-148. [CrossRef]
- Chiavarini M, Ostorero A, Naldini G, Fabiani R. Cancer risk in children and young adults (offspring) born after medically assisted reproduction: a systematic review and meta-analysis. J. 2019;2(4):430-448. [CrossRef]
- Luke B, Brown MB, Wantman E, et al. The risks of birth defects and childhood cancer with conception by assisted reproductive technology. Hum Reprod. Oct 31, 2022;37(11):2672-2689. [CrossRef] [Medline]
- Rios P, Herlemont P, Fauque P, et al. Medically assisted reproduction and risk of cancer among offspring. JAMA Netw Open. May 1, 2024;7(5):e249429. [CrossRef] [Medline]
- Spaan M, Pontesilli M, van den Belt-Dusebout AW, et al. Cancer risk in children, adolescents, and young adults conceived by ART in 1983–2011. Human Reproduction Open. Jun 1, 2023;2023(3):hoad027. [CrossRef]
- Wainstock T, Walfisch A, Shoham-Vardi I, et al. Fertility treatments and pediatric neoplasms of the offspring: results of a population-based cohort with a median follow-up of 10 years. Am J Obstet Gynecol. Mar 2017;216(3):e1-e14. [CrossRef]
- Weng SS, Huang YT, Huang YT, Li YP, Chien LY. Assisted reproductive technology and risk of childhood cancers. JAMA Netw Open. Aug 1, 2022;5(8):e2230157. [CrossRef] [Medline]
- Hargreave M, Jensen A, Hansen MK, et al. Association between fertility treatment and cancer risk in children. JAMA. Dec 10, 2019;322(22):2203-2210. [CrossRef] [Medline]
- Sargisian N, Lannering B, Petzold M, et al. Cancer in children born after frozen-thawed embryo transfer: a cohort study. PLoS Med. Sep 2022;19(9):e1004078. [CrossRef] [Medline]
- Terho AM, Tiitinen A, Martikainen H, Gissler M, Pelkonen S. Health of singletons born after frozen embryo transfer until early adulthood: a Finnish register study. Hum Reprod. Nov 24, 2022;37(12):2899-2907. [CrossRef]
- Page MJ, McKenzie JE, Bossuyt PM, et al. The PRISMA 2020 statement: an updated guideline for reporting systematic reviews. BMJ. Mar 29, 2021;372:n71. [CrossRef] [Medline]
- Tang X, Han YP, Chai YH, et al. Association of kidney function and brain health: a systematic review and meta-analysis of cohort studies. Ageing Res Rev. Dec 2022;82:101762. [CrossRef] [Medline]
- DerSimonian R, Laird N. Meta-analysis in clinical trials. Control Clin Trials. Sep 1986;7(3):177-188. [CrossRef] [Medline]
- Andrews JC, Schünemann HJ, Oxman AD, et al. GRADE guidelines: 15. Going from evidence to recommendation—determinants of a recommendation's direction and strength. J Clin Epidemiol. Jul 2013;66(7):726-735. [CrossRef] [Medline]
- Bruinsma F, Venn A, Lancaster P, Speirs A, Healy D. Incidence of cancer in children born after in-vitro fertilization. Hum Reprod. Mar 2000;15(3):604-607. [CrossRef]
- Bal MH, Harlev A, Sergienko R, et al. Possible association between in vitro fertilization technologies and offspring neoplasm. Fertil Steril. Jul 2021;116(1):105-113. [CrossRef] [Medline]
- Pinborg A, Loft A, Aaris Henningsen AK, Rasmussen S, Andersen AN. Infant outcome of 957 singletons born after frozen embryo replacement: the Danish National Cohort Study 1995-2006. Fertil Steril. Sep 2010;94(4):1320-1327. [CrossRef]
- Spector LG, Brown MB, Wantman E, et al. Association of in vitro fertilization with childhood cancer in the United States. JAMA Pediatr. Jun 1, 2019;173(6):e190392. [CrossRef] [Medline]
- Sundh KJ, Henningsen AKA, Källen K, et al. Cancer in children and young adults born after assisted reproductive technology: a Nordic cohort study from the Committee of Nordic ART and Safety (CoNARTaS). Hum Reprod. Sep 2014;29(9):2050-2057. [CrossRef] [Medline]
- Williams CL, Bunch KJ, Stiller CA, et al. Cancer risk among children born after assisted conception. N Engl J Med. Nov 7, 2013;369(19):1819-1827. [CrossRef] [Medline]
- Foix-L’Hélias L, Aerts I, Marchand L, et al. Are children born after infertility treatment at increased risk of retinoblastoma? Hum Reprod. Jul 2012;27(7):2186-2192. [CrossRef] [Medline]
- Rudant J, Amigou A, Orsi L, et al. Fertility treatments, congenital malformations, fetal loss, and childhood acute leukemia: the ESCALE study (SFCE). Pediatr Blood Cancer. Feb 2013;60(2):301-308. [CrossRef] [Medline]
- Ajrouche R, Rudant J, Orsi L, et al. Maternal reproductive history, fertility treatments and folic acid supplementation in the risk of childhood acute leukemia: the ESTELLE study. Cancer Causes Control. Oct 2014;25(10):1283-1293. [CrossRef]
- Munzer C, Menegaux F, Lacour B, et al. Birth-related characteristics, congenital malformation, maternal reproductive history and neuroblastoma: the ESCALE study (SFCE). Int J Cancer. May 15, 2008;122(10):2315-2321. [CrossRef] [Medline]
- Borgmann-Staudt A, Michael S, Sommerhaeuser G, et al. The use of assisted reproductive technology by European childhood cancer survivors. Curr Oncol. Aug 15, 2022;29(8):5748-5762. [CrossRef] [Medline]
- Farland LV, Liu CL, Diop H, et al. Hospitalizations up to 8 years following delivery in assisted reproductive technology-treated and subfertile women. Fertil Steril. Mar 2022;117(3):593-602. [CrossRef] [Medline]
- Chen M, Heilbronn LK. The health outcomes of human offspring conceived by assisted reproductive technologies (ART). J Dev Orig Health Dis. Aug 2017;8(4):388-402. [CrossRef]
- Doornbos ME, Maas SM, McDonnell J, Vermeiden JPW, Hennekam RCM. Infertility, assisted reproduction technologies and imprinting disturbances: a Dutch study. Hum Reprod. Sep 2007;22(9):2476-2480. [CrossRef] [Medline]
- Ludwig M, Katalinic A, Gross S, Sutcliffe A, Varon R, Horsthemke B. Increased prevalence of imprinting defects in patients with Angelman syndrome born to subfertile couples. J Med Genet. Apr 2005;42(4):289-291. [CrossRef] [Medline]
- Niemitz EL, Feinberg AP. Epigenetics and assisted reproductive technology: a call for investigation. Am J Hum Genet. Apr 2004;74(4):599-609. [CrossRef] [Medline]
- Dyer S, Chambers GM, de Mouzon J, et al. International Committee for Monitoring Assisted Reproductive Technologies world report: assisted reproductive technology 2008, 2009 and 2010. Hum Reprod. Jul 2016;31(7):1588-1609. [CrossRef] [Medline]
- Calhaz-Jorge C, De Geyter C, Kupka MS, et al. Assisted reproductive technology in Europe, 2013: results generated from European registers by ESHRE. Hum Reprod. Oct 1, 2017;32(10):1957-1973. [CrossRef]
- European IVF-Monitoring Consortium (EIM) for the European Society of Human Reproduction and Embryology (ESHRE), Wyns C, Bergh C, et al. ART in Europe, 2016: results generated from European registries by ESHRE. Hum Reprod Open. 2020;2020(3):hoaa032. [CrossRef] [Medline]
- Pelkonen S, Koivunen R, Gissler M, et al. Perinatal outcome of children born after frozen and fresh embryo transfer: the Finnish cohort study 1995-2006. Hum Reprod. Apr 2010;25(4):914-923. [CrossRef] [Medline]
- Maheshwari A, Pandey S, Amalraj Raja E, Shetty A, Hamilton M, Bhattacharya S. Is frozen embryo transfer better for mothers and babies? Can cumulative meta-analysis provide a definitive answer? Hum Reprod Update. Jan 1, 2018;24(1):35-58. [CrossRef] [Medline]
- Sha T, Yin X, Cheng W, Massey IY. Pregnancy-related complications and perinatal outcomes resulting from transfer of cryopreserved versus fresh embryos in vitro fertilization: a meta-analysis. Fertil Steril. Feb 2018;109(2):330-342. [CrossRef]
- Terho AM, Pelkonen S, Opdahl S, et al. High birth weight and large-for-gestational-age in singletons born after frozen compared to fresh embryo transfer, by gestational week: a Nordic register study from the CoNARTaS group. Hum Reprod. Mar 18, 2021;36(4):1083-1092. [CrossRef]
- Elias FTS, Weber-Adrian D, Pudwell J, et al. Neonatal outcomes in singleton pregnancies conceived by fresh or frozen embryo transfer compared to spontaneous conceptions: a systematic review and meta-analysis. Arch Gynecol Obstet. Jul 2020;302(1):31-45. [CrossRef] [Medline]
- Reigstad MM, Larsen IK, Myklebust TÅ, et al. Risk of cancer in children conceived by assisted reproductive technology. Pediatrics. Mar 2016;137(3):e20152061. [CrossRef] [Medline]
- European IVF-Monitoring Consortium (EIM) for the European Society of Human Reproduction and Embryology (ESHRE), Wyns C, De Geyter C, et al. ART in Europe, 2017: results generated from European registries by ESHRE. Hum Reprod Open. 2021;2021(3):hoab026. [CrossRef] [Medline]
- Marino JL, Moore VM, Willson KJ, et al. Perinatal outcomes by mode of assisted conception and sub-fertility in an Australian data linkage cohort. PLoS One. 2014;9(1):e80398. [CrossRef] [Medline]
- Lerner-Geva L, Boyko V, Ehrlich S, et al. Possible risk for cancer among children born following assisted reproductive technology in Israel. Pediatr Blood Cancer. Apr 2017;64(4):e26292. [CrossRef] [Medline]
- Wu Y, Deng Y, Wei B, et al. Global, regional, and national childhood cancer burden, 1990–2019: an analysis based on the Global Burden of Disease Study 2019. J Adv Res. Sep 2022;40:233-247. [CrossRef]
- Petridou ET, Sergentanis TN, Panagopoulou P, et al. In vitro fertilization and risk of childhood leukemia in Greece and Sweden. Pediatr Blood Cancer. Jun 2012;58(6):930-936. [CrossRef] [Medline]
- Schulz KF, Grimes DA. Case-control studies: research in reverse. Lancet. Feb 2002;359(9304):431-434. [CrossRef]
Abbreviations
ART: assisted reproductive technology |
FET: frozen embryo transfer |
fresh-ET: fresh embryo transfer |
GRADE: Grading of Recommendations Assessment, Development, and Evaluation |
ICSI: intracytoplasmic sperm injection |
INPLASY: International Platform of Registered Systematic Review and Meta-Analysis Protocols |
IUI: intrauterine insemination |
IVF: in vitro fertilization |
LGA: large for gestational age |
NOS: Newcastle-Ottawa Quality Assessment Scale |
OI: ovulation induction |
OR: odds ratio |
PICOS: Population, Intervention, Comparison, Outcomes, and Study Design |
PRISMA: Preferred Reporting Items for Systematic Reviews and Meta-Analyses |
RR: relative risk |
Edited by Naomi Cahill; submitted 27.08.24; peer-reviewed by Ana Clara Felix De Farias Santos, Ayad Palani, E Stern Judy; final revised version received 26.01.25; accepted 27.01.25; published 12.03.25.
Copyright© Gao Song, Cai-qiong Zhang, Zhong-ping Bai, Rong Li, Meng-qun Cheng. Originally published in JMIR Cancer (https://cancer.jmir.org), 12.3.2025.
This is an open-access article distributed under the terms of the Creative Commons Attribution License (https://creativecommons.org/licenses/by/4.0/), which permits unrestricted use, distribution, and reproduction in any medium, provided the original work, first published in JMIR Cancer, is properly cited. The complete bibliographic information, a link to the original publication on https://cancer.jmir.org/, as well as this copyright and license information must be included.