Key Points
-
The current prevalence rates of autism spectrum disorder (ASD) are about 1 in a 100, with a greater incidence in boys.
-
ASD has a strong genetic component, and new sequencing methods have allowed the identification of many rare and de novo mutations that can contribute to the condition.
-
Many of the genes associated with ASD encode for proteins that act at the synapse, which suggests that these might be potential points of intervention and therapeutic targets.
-
Induced pluripotent stem cells derived from individuals with ASD and animal models with construct and face validity provide an opportunity to identify cellular and molecular phenotypes associated with the condition.
-
Clinical development of therapeutics for ASD poses particular challenges with regard to identification of suitable end points, treatment of paediatric populations, lack of biomarkers for progression, and regulatory considerations.
-
Ongoing clinical trials for genetically defined neurodevelopmental disorders such as fragile X syndrome offer a valuable opportunity to learn about considerations for clinical development for ASD and related disorders.
Abstract
The rising rates of autism spectrum disorder (ASD) and the lack of effective medications to treat its core symptoms have led to an increased sense of urgency to identify therapies for this group of neurodevelopmental conditions. Developing drugs for ASD, however, has been challenging because of a limited understanding of its pathophysiology, difficulties in modelling the disease in vitro and in vivo, the heterogeneity of symptoms, and the dearth of prior experience in clinical development. In the past few years these challenges have been mitigated by considerable advances in our understanding of forms of ASD caused by single-gene alterations, such as fragile X syndrome and tuberous sclerosis. In these cases we have gained insights into the pathophysiological mechanisms underlying these conditions. In addition, they have aided in the development of animal models and compounds with the potential for disease modification in clinical development. Moreover, genetic studies are illuminating the molecular pathophysiology of ASD, and new tools such as induced pluripotent stem cells offer novel possibilities for drug screening and disease diagnostics. Finally, large-scale collaborations between academia and industry are starting to address some of the key barriers to developing drugs for ASD. Here, we propose a conceptual framework for drug discovery in ASD encompassing target identification, drug profiling and considerations for clinical trials in this novel area.
This is a preview of subscription content, access via your institution
Access options
Access to this article via ICE Institution of Civil Engineers is not available.
Subscribe to this journal
Receive 12 print issues and online access
$209.00 per year
only $17.42 per issue
Buy this article
- Purchase on SpringerLink
- Instant access to full article PDF
Prices may be subject to local taxes which are calculated during checkout
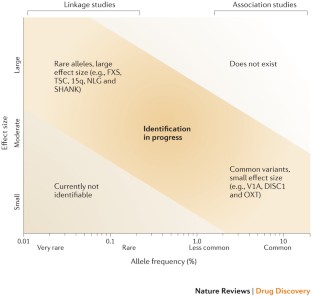
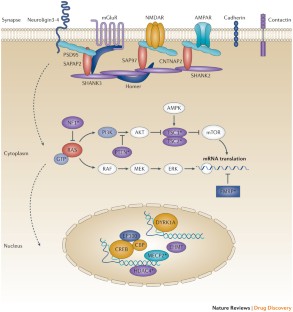
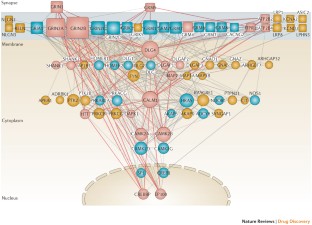
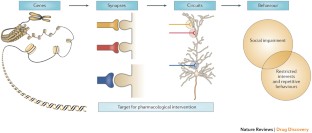
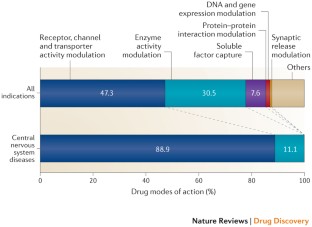
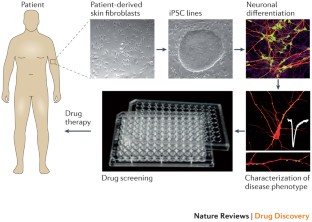
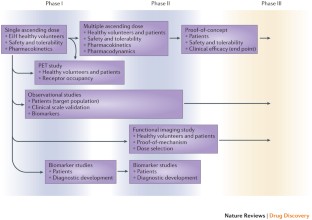
Similar content being viewed by others
References
Kanner, L. Autistic disturbances of affective contact. Nerv. Child. 2, 217–250 (1943).
Asperger, H. The psychically abnormal child, Wien Klin. Wochenschr. 51, 1314–1317 (1938).
Centers for Disease Control and Prevention. Prevalence of autism spectrum disorders — Autism and Developmental Disabilities Monitoring Network, 14 sites, United States, 2008. MMWR Surveill. Summ. 61, 1–19 (2012).
Politte, L. C. & McDougle, C. J. Atypical antipsychotics in the treatment of children and adolescents with pervasive developmental disorders. Psychopharmacology (Berl). 2013 (dx.http://dx.doi.org/10.1007/s00213-013-3068-y).
Ehninger, D. et al. Reversal of learning deficits in a Tsc2+/− mouse model of tuberous sclerosis. Nature Med. 14, 843–848 (2008).
Michalon, A. et al. Chronic pharmacological mGlu5 inhibition corrects fragile X in adult mice. Neuron 74, 49–56 (2012).
Costa, R. M. et al. Mechanism for the learning deficits in a mouse model of neurofibromatosis type 1. Nature 415, 526–530 (2002).
Tropea, D. et al. Partial reversal of Rett syndrome-like symptoms in MeCP2 mutant mice. Proc. Natl Acad. Sci. USA 106, 2029–2034 (2009).
Meikle, L. et al. Response of a neuronal model of tuberous sclerosis to mammalian target of rapamycin (mTOR) inhibitors: effects on mTORC1 and Akt signaling lead to improved survival and function. J. Neurosci. 28, 5422–5432 (2008).
Ronald, A. & Hoekstra, R. A. Autism spectrum disorders and autistic traits: a decade of new twin studies. Am. J. Med. Genet. B Neuropsychiatr. Genet. 156B, 255–274 (2011).
Kumar, R. A. & Christian, S. L. Genetics of autism spectrum disorders. Curr. Neurol. Neurosci. Rep. 9, 188–197 (2009).
Betancur, C. Etiological heterogeneity in autism spectrum disorders: more than 100 genetic and genomic disorders and still counting. Brain Res. 1380, 42–77 (2011).
Marshall, C. R. & Scherer, S. W. Detection and characterization of copy number variation in autism spectrum disorder. Methods Mol. Biol. 838, 115–135 (2012).
Kong, A. et al. Rate of de novo mutations and the importance of father's age to disease risk. Nature 488, 471–475 (2012).
Gibson, G. Rare and common variants: twenty arguments. Nature Rev. Genet. 13, 135–145 (2012).
Barabasi, A. L., Gulbahce, N. & Loscalzo, J. Network medicine: a network-based approach to human disease. Nature Rev. Genet. 12, 56–68 (2011).
Peca, J. & Feng, G. Cellular and synaptic network defects in autism. Curr. Opin. Neurobiol. 22, 866–872 (2012).
Etherton, M. R., Tabuchi, K., Sharma, M., Ko, J. & Sudhof, T. C. An autism-associated point mutation in the neuroligin cytoplasmic tail selectively impairs AMPA receptor-mediated synaptic transmission in hippocampus. EMBO J. 30, 2908–2919 (2011).
Etherton, M. et al. Autism-linked neuroligin-3 R451C mutation differentially alters hippocampal and cortical synaptic function. Proc. Natl Acad. Sci. USA 108, 13764–13769 (2011).
Kelleher, R. J., 3rd & Bear, M. F. The autistic neuron: troubled translation? Cell 135, 401–406 (2008).
van Bokhoven, H. Genetic and epigenetic networks in intellectual disabilities. Annu. Rev. Genet. 45, 81–104 (2011).
Schneider, A., Hagerman, R. J. & Hessl, D. Fragile X syndrome — from genes to cognition. Dev. Disabil Res. Rev. 15, 333–342 (2009).
Krueger, D. D. & Bear, M. F. Toward fulfilling the promise of molecular medicine in fragile X syndrome. Annu. Rev. Med. 62, 411–429 (2011).
Dölen, G. et al. Correction of fragile X syndrome in mice. Neuron 56, 955–962 (2007).
Hur, E. M. & Zhou, F. Q. GSK3 signalling in neural development. Nature Rev. Neurosci. 11, 539–551 (2010).
Bilousova, T. V. et al. Minocycline promotes dendritic spine maturation and improves behavioural performance in the fragile X mouse model. J. Med. Genet. 46, 94–102 (2009).
Yuskaitis, C. J. et al. Lithium ameliorates altered glycogen synthase kinase-3 and behavior in a mouse model of fragile X syndrome. Biochem. Pharmacol. 79, 632–646 (2010).
Mines, M. A., Yuskaitis, C. J., King, M. K., Beurel, E. & Jope, R. S. GSK3 influences social preference and anxiety-related behaviors during social interaction in a mouse model of fragile X syndrome and autism. PLoS ONE 5, e9706 (2010).
Paluszkiewicz, S. M., Martin, B. S. & Huntsman, M. M. Fragile X syndrome: the GABAergic system and circuit dysfunction. Dev. Neurosci. 33, 349–364 (2011).
Henderson, C. et al. Reversal of disease-related pathologies in the fragile X mouse model by selective activation of GABAB receptors with arbaclofen. Sci. Transl. Med. 4, 152ra128 (2012).
Curatolo, P., Bombardieri, R. & Jozwiak, S. Tuberous sclerosis. Lancet 372, 657–668 (2008).
Tavazoie, S. F., Alvarez, V. A., Ridenour, D. A., Kwiatkowski, D. J. & Sabatini, B. L. Regulation of neuronal morphology and function by the tumor suppressors Tsc1 and Tsc2. Nature Neurosci. 8, 1727–1734 (2005).
Meikle, L. et al. A mouse model of tuberous sclerosis: neuronal loss of Tsc1 causes dysplastic and ectopic neurons, reduced myelination, seizure activity, and limited survival. J. Neurosci. 27, 5546–5558 (2007).
Davies, D. M. et al. Sirolimus therapy for angiomyolipoma in tuberous sclerosis and sporadic lymphangioleiomyomatosis: a phase 2 trial. Clin. Cancer Res. 17, 4071–4081 (2011).
Bissler, J. J. et al. Sirolimus for angiomyolipoma in tuberous sclerosis complex or lymphangioleiomyomatosis. N. Engl. J. Med. 358, 140–151 (2008).
Dabora, S. L. et al. Multicenter phase 2 trial of sirolimus for tuberous sclerosis: kidney angiomyolipomas and other tumors regress and VEGF- D levels decrease. PLoS ONE 6, e23379 (2011).
Silverman, J. L. et al. Negative allosteric modulation of the mGluR5 receptor reduces repetitive behaviors and rescues social deficits in mouse models of autism. Sci. Transl. Med. 4, 131ra51 (2012).
Auerbach, B. D., Osterweil, E. K. & Bear, M. F. Mutations causing syndromic autism define an axis of synaptic pathophysiology. Nature 480, 63–68 (2011).
Spooren, W., Lindemann, L., Ghosh, A. & Santarelli, L. Synapse dysfunction in autism: a molecular medicine approach to drug discovery in neurodevelopmental disorders. Trends Pharm. Sci 33, 669–684 (2012).
Mabb, A. M., Judson, M. C., Zylka, M. J. & Philpot, B. D. Angelman syndrome: insights into genomic imprinting and neurodevelopmental phenotypes. Trends Neurosci. 34, 293–303 (2012).
Kim, J. E. et al. Investigating synapse formation and function using human pluripotent stem cell-derived neurons. Proc. Natl Acad. Sci. USA 108, 3005–3010 (2011).
Dimos, J. T. et al. Induced pluripotent stem cells generated from patients with ALS can be differentiated into motor neurons. Science 321, 1218–1221 (2008).
Marchetto, M. C. et al. A model for neural development and treatment of Rett syndrome using human induced pluripotent stem cells. Cell 143, 527–539 (2010).
Pasca, S. P. et al. Using iPSC-derived neurons to uncover cellular phenotypes associated with Timothy syndrome. Nature Med. 17, 1657–1662 (2011).
McFarlane, H. G. et al. Autism-like behavioral phenotypes in BTBR T+tf/J mice. Genes Brain Behav. 7, 152–163 (2008).
Roullet, F. I., Lai, J. K. & Foster, J. A. In utero exposure to valproic acid and autism — a current review of clinical and animal studies. Neurotoxicol. Teratol. 36, 47–56 (2013).
Silverman, J. L., Yang, M., Lord, C. & Crawley, J. N. Behavioural phenotyping assays for mouse models of autism. Nature Rev. Neurosci. 11, 490–502 (2010).
Crawley, J. N. Translational animal models of autism and neurodevelopmental disorders. Dialogues Clin. Neurosci. 14, 293–305 (2012).
Scattoni, M. L., Ricceri, L. & Crawley, J. N. Unusual repertoire of vocalizations in adult BTBR T+tf/J mice during three types of social encounters. Genes Brain Behav. 10, 44–56 (2011).
Young, D. M., Schenk, A. K., Yang, S. B., Jan, Y. N. & Jan, L. Y. Altered ultrasonic vocalizations in a tuberous sclerosis mouse model of autism. Proc. Natl Acad. Sci. USA 107, 11074–11079 (2010).
Roy, S., Watkins, N. & Heck, D. Comprehensive analysis of ultrasonic vocalizations in a mouse model of fragile X syndrome reveals limited, call type specific deficits. PLoS ONE 7, e44816 (2012).
Rotschafer, S. E., Trujillo, M. S., Dansie, L. E., Ethell, I. M. & Razak, K. A. Minocycline treatment reverses ultrasonic vocalization production deficit in a mouse model of fragile X syndrome. Brain Res. 1439, 7–14 (2012).
Constantino, J. N. et al. The factor structure of autistic traits. J. Child Psychol. Psychiatry 45, 719–726 (2004).
Jacquemont, S. et al. Epigenetic modification of the FMR1 gene in fragile X syndrome is associated with differential response to the mGluR5 antagonist AFQ056. Sci. Transl. Med. 3, 64ra1 (2011).
Berry-Kravis, E. M. et al. Effects of STX209 (arbaclofen) on neurobehavioral function in children and adults with fragile X syndrome: a randomized, controlled, Phase 2 trial. Sci. Transl. Med. 4, 152ra127 (2012).
Berry-Kravis, E. et al. Open-label treatment trial of lithium to target the underlying defect in fragile X syndrome. J. Dev. Behav. Pediatr. 29, 293–302 (2008).
Paribello, C. et al. Open-label add-on treatment trial of minocycline in fragile X syndrome. BMC Neurol. 10, 91 (2010).
Leigh, M. J. et al. A randomized double-blind, placebo-controlled trial of minocycline in children and adolescents with fragile X syndrome. J. Dev. Behav. Pediatr. 34, 147–155 (2013).
Pelphrey, K. A., Shultz, S., Hudac, C. M. & Vander Wyk, B. C. Research review: Constraining heterogeneity: the social brain and its development in autism spectrum disorder. J. Child Psychol. Psychiatry 52, 631–644 (2011).
Farzin, F., Scaggs, F., Hervey, C., Berry-Kravis, E. & Hessl, D. Reliability of eye tracking and pupillometry measures in individuals with fragile X syndrome. J. Autism Dev. Disord. 41, 1515–1522 (2011).
Zink, C. F. & Meyer-Lindenberg, A. Human neuroimaging of oxytocin and vasopressin in social cognition. Horm. Behav. 61, 400–409 (2012).
Andari, E. et al. Promoting social behavior with oxytocin in high-functioning autism spectrum disorders. Proc. Natl Acad. Sci. USA 107, 4389–4394 (2010).
Sansone, S. M. et al. Psychometric study of the aberrant behavior checklist in fragile X syndrome and implications for targeted treatment. J. Autism Dev. Disord. 42, 1377–1392 (2012).
Veenstra-VanderWeele, J. et al. Randomized, controlled, Phase 2 trial of STX209 for social function in ASD. International Society for Autism Research [online], (2013).
Chakrabarti, S. & Fombonne, E. Pervasive developmental disorders in preschool children: confirmation of high prevalence. Am. J. Psychiatry 162, 1133–1141 (2005).
Simonoff, E. et al. Psychiatric disorders in children with autism spectrum disorders: prevalence, comorbidity, and associated factors in a population-derived sample. J. Am. Acad. Child Adolesc. Psychiatry 47, 921–929 (2008).
Mattila, M. L. et al. Comorbid psychiatric disorders associated with Asperger syndrome/high-functioning autism: a community- and clinic-based study. J. Autism Dev. Disord. 40, 1080–1093 (2010).
Boyd, B. A. et al. Sensory features and repetitive behaviors in children with autism and developmental delays. Autism Res. 3, 78–87 (2010).
Chez, M. G. et al. Frequency of epileptiform EEG abnormalities in a sequential screening of autistic patients with no known clinical epilepsy from 1996 to 2005. Epilepsy Behav. 8, 267–271 (2006).
Buie, T. et al. Evaluation, diagnosis, and treatment of gastrointestinal disorders in individuals with ASDs: a consensus report. Pediatrics 125 (Suppl. 1), 1–18 (2010).
Stefanatos, G. A. Regression in autistic spectrum disorders. Neuropsychol. Rev. 18, 305–319 (2008).
Fombonne, E. Epidemiology of pervasive developmental disorders. Pediatr. Res. 65, 591–598 (2009).
Goldman, M. The innovative medicines initiative: a European response to the innovation challenge. Clin. Pharmacol. Ther. 91, 418–425 (2012).
Laverty, H., Gunn, M. & Goldman, M. Improving R&D productivity of pharmaceutical companies through public–private partnership: experiences from the Innovative Medicines Initiative. Expert Rev. Pharmacoecon. Outcomes Res. 12, 545–548 (2012).
Murphy, D. & Spooren, W. EU-AIMS: a boost to autism research. Nature Rev. Drug Discov. 11, 815–816 (2012).
Klei, L. et al. Common genetic variants, acting additively, are a major source of risk for autism. Mol. Autism 3, 9 (2012).
Neale, B. M. et al. Patterns and rates of exonic de novo mutations in autism spectrum disorders. Nature 485, 242–245 (2012).
Michaelson, J. J. et al. Whole-genome sequencing in autism identifies hot spots for de novo germline mutation. Cell 151, 1431–1442 (2012).
Manolio, T. A. et al. Finding the missing heritability of complex diseases. Nature 461, 747–753 (2009).
Girirajan, S. et al. Phenotypic heterogeneity of genomic disorders and rare copy-number variants. N. Engl. J. Med. 367, 1321–1331 (2012).
Ollikainen, M. & Craig, J. M. Epigenetic discordance at imprinting control regions in twins. Epigenomics 3, 295–306 (2011).
McCarthy, M. I. et al. Genome-wide association studies for complex traits: consensus, uncertainty and challenges. Nature Rev. Genet. 9, 356–369 (2008).
Betancur, C., Sakurai, T. & Buxbaum, J. D. The emerging role of synaptic cell-adhesion pathways in the pathogenesis of autism spectrum disorders. Trends Neurosci. 32, 402–412 (2009).
Franceschini, A. et al. STRING v9.1: protein–protein interaction networks, with increased coverage and integration. Nucleic Acids Res. 41, D808–D815 (2013).
Darnell, J. C. et al. FMRP stalls ribosomal translocation on mRNAs linked to synaptic function and autism. Cell 146, 247–261 (2011).
Saito, R. et al. A travel guide to Cytoscape plugins. Nature Methods 9, 1069–1076 (2012).
Acknowledgements
The authors would like to thank M. Ebelin and J. Gottowick for their excellent support with the generation of the synaptic network for figure 3 and with information retrieval from drug databases for figure 5.
Author information
Authors and Affiliations
Corresponding author
Ethics declarations
Competing interests
All authors are or were (A.M.) full time employees at F. Hoffmann-La Roche.
Glossary
- Face validity
-
The extent to which an animal model's phenotype resembles human symptoms (e.g., an Alzheimer's disease model that progressively develops amyloid plaques and memory impairment).
- Construct validity
-
The extent to which an animal model has the same aetiology and underlying mechanism as the human disorder (e.g., an Alzheimer's disease model generated by introducing a human presenilin mutation in the mouse)
- RAS–MAPK–ERK pathway
-
RAS–mitogen-activated protein kinase (MAPK)–extracellular signal-regulated kinase (ERK) pathway.
- PI3K–AKT–mTOR pathway
-
Phosphoinositide 3-kinase (PI3K)–AKT–mammalian target of rapamycin (mTOR) pathway.
- CREB pathway
-
Cyclic AMP-response element-binding protein (CREB) pathway.
- Long-term potentiation
-
(LTP). A persistent strengthening of synaptic transmission in response to strong, correlated input.
- Long-term depression
-
(LTD). The converse of LTP; in LTD there is a long-lasting and activity-dependent decrease in synaptic efficacy.
- Predictive validity
-
An animal model's ability to help identify drugs with potential therapeutic value in humans (e.g., antidepressant drugs reliably stimulate escape behaviours in the forced swim test, so the assay predicts antidepressant efficacy even if it does not measure depression).
- Aberrant Behaviour Checklist
-
(ABC). A symptom checklist developed to assess problem behaviours of children and adults with severe intellectual disability, mapping behaviours into 5 subscales: irritability and agitation, lethargy and social withdrawal, stereotypical behaviour, hyperactivity, and inappropriate speech.
- Social Responsiveness Scale
-
(SRS). A scale for quantitative measure of autism spectrum symptoms as they occur in natural social settings, specifically for social impairments such as social awareness, social information processing, capacity for reciprocal social communication, social anxiety/avoidance, and autistic preoccupations and traits. The SRS generates scores for five subscales: receptive, cognitive, expressive, and motivational aspects of social behaviour, as well as autistic preoccupations.
- Vineland Adaptive Behaviour Scale
-
(VABS). A semi-structured interview instrument that measures adaptive behaviour in children and adults, covering major personal and social skills required for everyday living. It is commonly used to support the diagnosis of intellectual and developmental disabilities including autism. Five major domains (including specific subdomains) are assessed, including communication, daily living skills, socialization, motor skills and maladaptive behaviour.
- Repeatable Battery for the Assessment of Neuropsychological Status
-
(RBANS). A brief neuropsychological battery that assesses cognitive decline or improvement, used as a neuropsychological screening battery for younger patients (down to 12 years). It tests five cognitive domains: immediate memory, visuospatial/constructional, language, attention and delayed memory.
- Visual Analogue Scale
-
An instrument to assess subjective characteristics or attitudes that cannot be directly measured, in which respondents specify their level of agreement to a statement by indicating a position along a continuous line between two end points.
- Clinical Global Impression (CGI) scale
-
A commonly used summary measure of a patients' global functioning. The CGI Severity is a seven-point categorical scale (from normal to extremely ill) that rates the severity of the disease at the time of assessment in comparison with past experience of patients with the same diagnosis. The CGI Improvement is a seven-point categorical scale (from very much improved to very much worse) that measures change in patient status from baseline in response to an intervention.
Rights and permissions
About this article
Cite this article
Ghosh, A., Michalon, A., Lindemann, L. et al. Drug discovery for autism spectrum disorder: challenges and opportunities. Nat Rev Drug Discov 12, 777–790 (2013). https://doi.org/10.1038/nrd4102
Published:
Issue Date:
DOI: https://doi.org/10.1038/nrd4102